Scanning Resonator Microscopy (SRM)
Scanning Resonator Microscopy Integrating Phase Sensitive Detection
(Applied Optics 2017, 56(35), 9716-9723)
Scanning resonator microscopy (SRM) is a scanning probe technique that uses a small, optical resonator attached to the end of a conventional atomic force microscopy (AFM) cantilever to simultaneously measure optical and topography properties of sample surfaces. In SRM, whispering gallery mode (WGM) resonances excited in the attached optical resonator shift in response to changes in surface refractive index (RI), providing a mechanism for mapping RI with high spatial resolution. In our initial report, the SRM tip was excited with a fixed excitation wavelength during sample scanning, which limits the approach. An improved method based on a wavelength modulation coupled with phase sensitive detection is reported here. This results in real-time characterization of WGM spectral shifts while eliminating complications arising from measurements based solely on signal intensity. This improved approach, combined with a modified tip design enabling integration of smaller resonators, is shown to enhance signal-to-noise and lead to sub-100 nm spatial resolution in the SRM optical image. The improved capabilities are demonstrated through measurements on thin dielectric and polymer films.
SRM Tip and Scheme
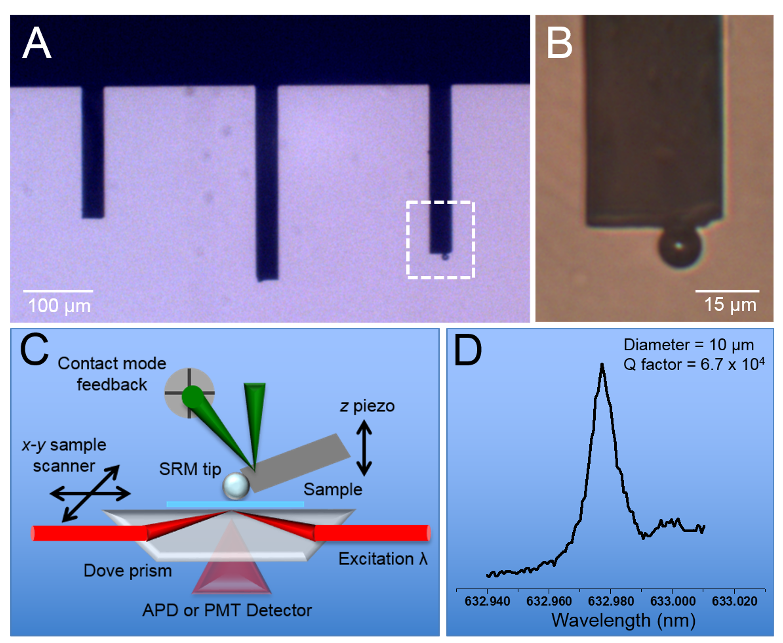
SRM Topography and Optical Images
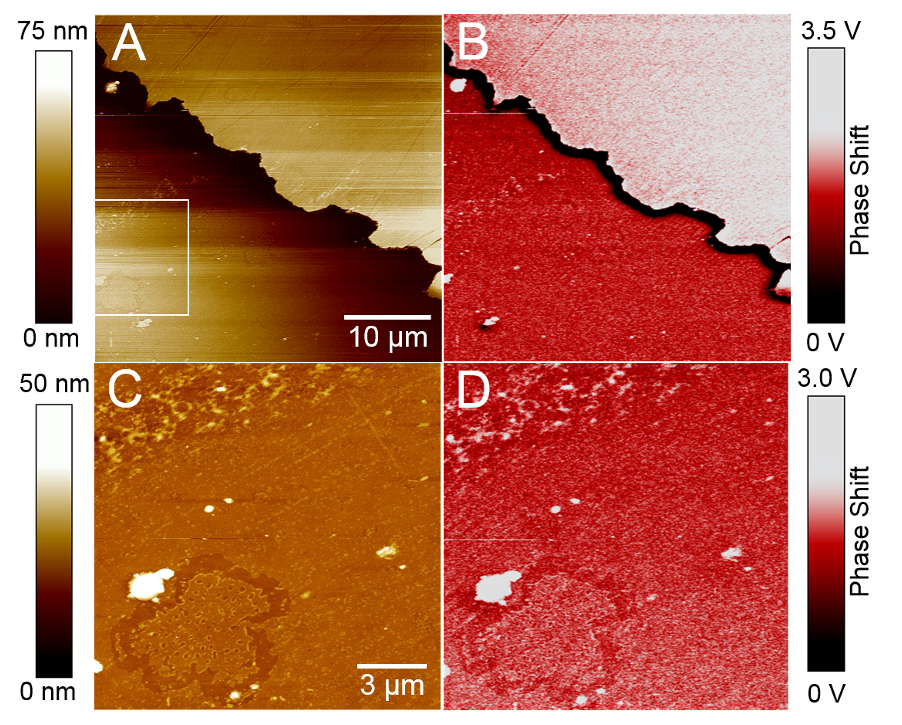
SRM topography and Optical Images
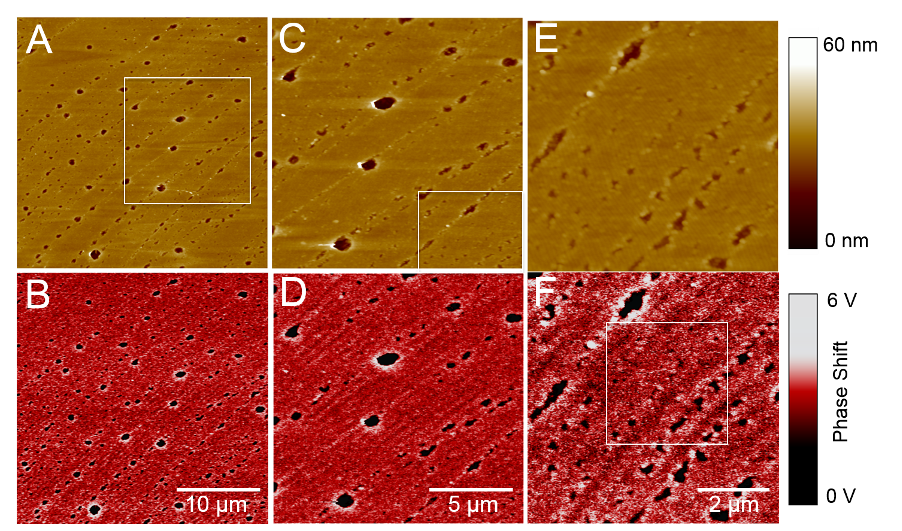
Scanning Resonator Microscopy: Integrating Whispering Gallery Mode Sensing with Atomic Force Microscopy
(ACS Photonics 2015, 2(6), 699-706)
Scanning resonator microscopy (SRM) is developed to integrate whispering gallery mode (WGM) sensing with atomic force microscopy (AFM). The hybrid technique combines the exquisite refractive index sensing of whispering gallery mode resonators with the topography mapping capabilities of AFM. A 45 μm diameter barium titanate microsphere is attached to the end of a conventional AFM cantilever and acts as both a WGM resonator and stylus for mapping surface topography. Calibration plots, taken in contact-mode feedback, show that the WGM spectrum responds to changes in both solution and substrate refractive index. SRM imaging of a glass substrate reveals changes in surface refractive index that correspond to a small, 36 nm high feature measured simultaneously in the contact-mode topography image. Spectral measurements confirm that the contrast arises from refractive index changes and not coupling with sample topography, thus validating the approach. Additional measurements on thin polymer films and protein coated surfaces are presented and discussed in terms of possible areas of application for SRM.
SRM Tip and Apparatus
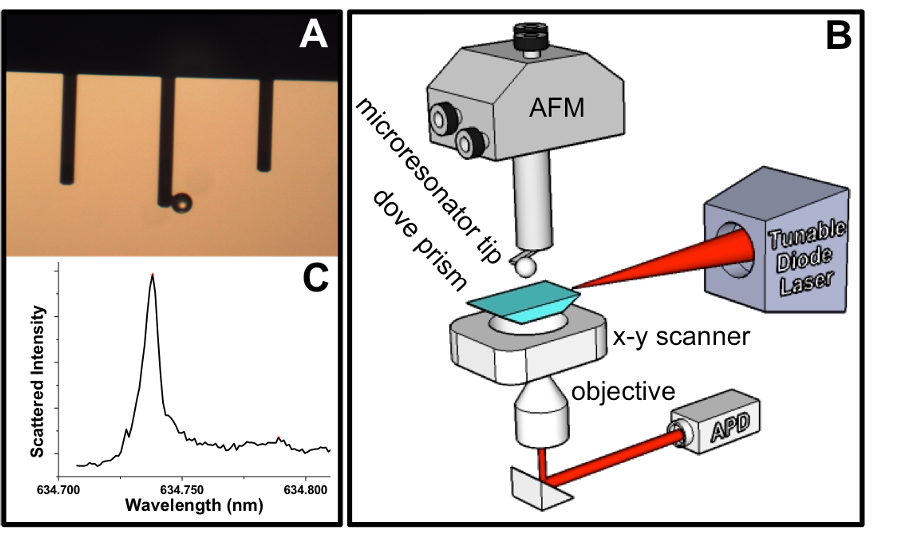
SRM Images and Spectral Shifts
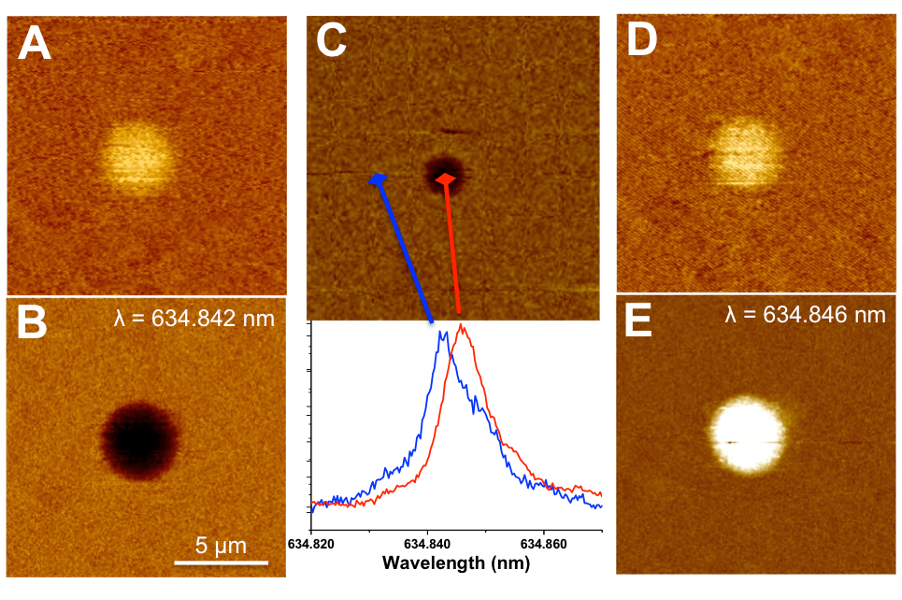